Specialty lab water treatment requirements
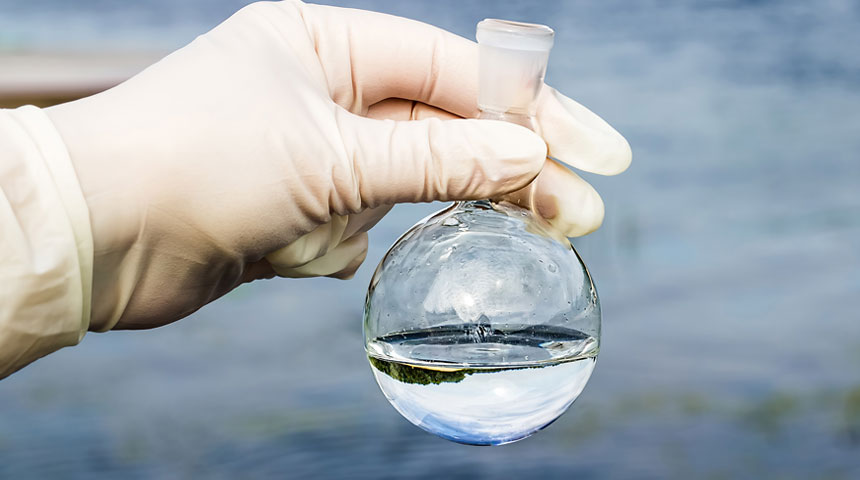
The Crucial Role of Lab Water Purity in Scientific Research and Public Health

In the realm of scientific research, the pursuit of knowledge and innovation hinges on the meticulous execution of experiments and analyses. The foundation of these endeavors lies in the quality of the reagents and materials employed, with lab water purity emerging as a paramount factor. This is particularly true in fields like molecular biology, cell culture, chromatography, and analytical chemistry, where even trace impurities can significantly impact experimental outcomes and compromise the validity of research findings. The implications extend beyond the confines of the laboratory, as the integrity of scientific data directly influences the development of new drugs, therapies, and technologies that impact public health and well-being.
The significance of lab water purity is underscored by its role in a wide range of critical applications. In molecular biology, for instance, water purity is essential for the accurate amplification of DNA and RNA using polymerase chain reaction (PCR) techniques. Contamination with even minute amounts of DNases or RNases can degrade nucleic acids, rendering PCR results unreliable and jeopardizing downstream analyses. Similarly, in cell culture, the presence of impurities in water can disrupt cell growth, morphology, and function, leading to inaccurate experimental data and compromised research findings.
The importance of lab water purity extends to the field of chromatography, a technique widely used for separating and analyzing complex mixtures. Impurities in water can interfere with the separation process, leading to inaccurate results and misinterpretations. This is particularly critical in analytical chemistry, where precise measurements are essential for determining the composition and purity of substances. The impact of water purity extends beyond the laboratory, influencing the development of new drugs, therapies, and technologies that directly impact public health.
The need for high-purity water in scientific research is not merely a matter of academic curiosity. It has profound implications for public health engineering, a field dedicated to ensuring the safety and quality of water supplies for human consumption. Water treatment processes, including purification and disinfection, rely on accurate analytical techniques that require high-purity water to ensure reliable results. The integrity of water quality testing is paramount in safeguarding public health, as it enables the identification and mitigation of potential contaminants that could pose health risks.
The use of high-purity water in public health engineering extends beyond water quality testing. It plays a crucial role in the production of disinfectants and other water treatment chemicals. The purity of these chemicals is essential for their effectiveness in eliminating harmful microorganisms and ensuring the safety of drinking water. Furthermore, high-purity water is used in the manufacturing of medical devices and pharmaceuticals, where contamination can have serious consequences for patient health.
The demand for high-purity water in scientific research and public health engineering has driven the development of sophisticated water treatment systems. These systems employ a range of technologies, including reverse osmosis, ion exchange, and distillation, to remove impurities and produce water that meets the stringent requirements of various applications. The choice of water treatment technology depends on the specific requirements of the application, the nature of the impurities present, and the desired level of purity.
The availability of high-quality lab water is essential for ensuring the accuracy and reliability of scientific research and the safety of public water supplies. As the demand for high-purity water continues to grow, it is imperative to invest in advanced water treatment technologies and to implement rigorous quality control measures to ensure that the water used in these critical applications meets the highest standards of purity.
The Crucial Role of Water Quality in Laboratory Operations
In the realm of scientific research and analysis, laboratories serve as the bedrock of innovation and discovery. From pharmaceutical development to environmental monitoring, the accuracy and reliability of laboratory results are paramount. A critical factor underpinning the integrity of these results is the quality of the water used in various laboratory procedures. Water, often considered a ubiquitous and innocuous substance, can harbor impurities that can significantly impact experimental outcomes, leading to inaccurate data, compromised research, and potentially flawed conclusions. This underscores the critical importance of water treatment in laboratory settings, ensuring that the water used meets the stringent purity requirements demanded by specific applications.
The impact of water quality on laboratory operations extends far beyond the realm of scientific accuracy. It directly influences the efficiency and cost-effectiveness of laboratory workflows. Contaminated water can lead to equipment malfunctions, clogging of instruments, and the need for frequent maintenance, all of which contribute to downtime and increased operational expenses. Furthermore, the use of untreated water can pose significant safety risks to laboratory personnel, potentially exposing them to harmful contaminants. This underscores the need for a comprehensive approach to water treatment in laboratories, encompassing not only the technical aspects of purification but also the safety and regulatory considerations that ensure a secure and compliant laboratory environment.
The demand for high-purity water in laboratories has spurred the development of sophisticated water treatment systems designed to remove a wide range of contaminants. These systems employ a combination of physical, chemical, and biological processes to achieve the desired level of purity. The specific treatment methods employed vary depending on the intended application and the nature of the contaminants present in the source water. For instance, laboratories conducting sensitive analytical procedures, such as chromatography or mass spectrometry, require water with extremely low levels of dissolved solids, organic compounds, and microbial contamination. In contrast, laboratories performing routine procedures may require water with a lower level of purity, but still exceeding the standards set by regulatory bodies.
Understanding the Spectrum of Water Purity Requirements
The purity requirements for laboratory water are often categorized into different grades, each defined by specific limits for contaminants. These grades are typically designated by acronyms, such as Type I, Type II, and Type III, with Type I representing the highest level of purity and Type III the lowest. The specific requirements for each grade are outlined in industry standards, such as the International Organization for Standardization (ISO) 3696 and the American Society for Testing and Materials (ASTM) D1193. These standards provide a framework for ensuring that laboratory water meets the necessary purity levels for various applications.
Type I water, often referred to as ultrapure water, is the gold standard for laboratory applications requiring the highest level of purity. It is typically used in sensitive analytical techniques, such as high-performance liquid chromatography (HPLC), gas chromatography (GC), and mass spectrometry (MS). Type I water is characterized by extremely low levels of dissolved solids, organic compounds, and microbial contamination. It is often produced using a multi-stage purification process that includes reverse osmosis, ion exchange, and ultrafiltration. The specific purification steps employed may vary depending on the specific requirements of the application.
Type II water, also known as reagent-grade water, is suitable for a wider range of laboratory applications, including cell culture, molecular biology, and general laboratory procedures. It typically has a lower level of purity than Type I water, but still meets the requirements for most laboratory procedures. Type II water is often produced using a combination of reverse osmosis and ion exchange. It may also undergo additional purification steps, such as activated carbon filtration or UV sterilization, to remove specific contaminants.
Type III water, also known as purified water, is the least pure grade of laboratory water. It is typically used for general laboratory procedures, such as washing glassware and preparing solutions. Type III water is often produced using a single-stage purification process, such as reverse osmosis or distillation. It may contain higher levels of dissolved solids and organic compounds than Type I or Type II water, but it is still suitable for many laboratory applications.
The Importance of Water Treatment in Public Health Engineering
The role of water treatment in public health engineering is paramount, ensuring the safety and quality of drinking water for entire populations. Public health engineers are responsible for designing, constructing, and operating water treatment plants that remove harmful contaminants from raw water sources, making it safe for human consumption. This process involves a series of steps, including coagulation, flocculation, sedimentation, filtration, and disinfection. Each step targets specific contaminants, ensuring that the final product meets the stringent standards set by regulatory agencies.
Coagulation and flocculation are the initial steps in the water treatment process, aimed at removing suspended solids from the raw water. Coagulation involves adding chemicals, such as aluminum sulfate or ferric chloride, to the water, causing small particles to clump together. Flocculation then uses gentle mixing to encourage these clumps, known as flocs, to grow larger and settle out of the water. Sedimentation follows, allowing the heavier flocs to settle to the bottom of the treatment tank, forming a sludge that is removed from the system.
Filtration is the next step in the water treatment process, removing any remaining suspended solids and other contaminants. Sand filters are commonly used, with layers of sand of varying sizes to trap particles of different sizes. Other filtration methods, such as membrane filtration, may also be employed to remove smaller particles and microorganisms. Disinfection is the final step in the water treatment process, ensuring that any remaining harmful microorganisms are killed. Chlorination is the most common disinfection method, using chlorine gas or sodium hypochlorite to kill bacteria and viruses. Other disinfection methods, such as ozonation or ultraviolet (UV) radiation, may also be used.
The effectiveness of water treatment in public health engineering is crucial for preventing waterborne diseases and protecting public health. Contaminated water can transmit a wide range of diseases, including cholera, typhoid fever, and dysentery. By removing harmful contaminants from the water supply, water treatment plants play a vital role in safeguarding public health and ensuring the well-being of communities.
The Impact of Water Quality on Laboratory Research
The quality of water used in laboratory research can have a profound impact on the accuracy and reliability of experimental results. Contaminated water can introduce impurities that interfere with chemical reactions, affect cell growth, and alter the properties of materials. This can lead to inaccurate data, compromised research, and potentially flawed conclusions. For example, in analytical chemistry, the presence of dissolved solids in water can interfere with the separation and detection of analytes, leading to inaccurate measurements. In cell culture, contaminated water can introduce microorganisms that can contaminate cell cultures, leading to unreliable results.
The impact of water quality on laboratory research is not limited to the accuracy of experimental results. It can also affect the efficiency and cost-effectiveness of laboratory workflows. Contaminated water can lead to equipment malfunctions, clogging of instruments, and the need for frequent maintenance, all of which contribute to downtime and increased operational expenses. For example, the presence of dissolved solids in water can lead to the formation of scale on laboratory equipment, reducing its efficiency and requiring frequent cleaning. In addition, contaminated water can pose significant safety risks to laboratory personnel, potentially exposing them to harmful contaminants.
The Role of Water Treatment in Ensuring Laboratory Safety
Water treatment plays a crucial role in ensuring laboratory safety by removing contaminants that can pose health risks to laboratory personnel. Contaminated water can contain a wide range of harmful substances, including bacteria, viruses, heavy metals, and organic compounds. These contaminants can be inhaled, ingested, or absorbed through the skin, leading to a variety of health problems. For example, exposure to bacteria or viruses in contaminated water can cause respiratory infections, gastrointestinal illnesses, and skin infections. Exposure to heavy metals can lead to neurological damage, kidney failure, and cancer. Exposure to organic compounds can cause a range of health problems, including liver damage, reproductive problems, and cancer.
Water treatment systems are designed to remove these contaminants, ensuring that the water used in laboratories is safe for personnel. The specific treatment methods employed vary depending on the nature of the contaminants present in the source water and the specific safety requirements of the laboratory. For example, laboratories handling hazardous materials may require more stringent water treatment to remove specific contaminants that could pose a risk to personnel. In addition to removing contaminants, water treatment systems can also help to prevent the growth of microorganisms in laboratory water systems, further reducing the risk of contamination.
The Importance of Water Treatment in Laboratory Compliance
Water treatment is essential for ensuring laboratory compliance with regulatory standards. Regulatory agencies, such as the Food and Drug Administration (FDA) and the Environmental Protection Agency (EPA), set standards for the quality of water used in laboratories. These standards are designed to ensure that laboratory water meets the requirements for specific applications and to protect the health of laboratory personnel. Laboratories must comply with these standards to avoid fines and other penalties.
Water treatment systems play a crucial role in ensuring laboratory compliance by providing a reliable source of high-quality water that meets regulatory standards. The specific treatment methods employed must be validated to ensure that they effectively remove contaminants to the required levels. Laboratories must also maintain records of their water treatment processes, including the results of water quality testing, to demonstrate compliance with regulatory requirements. Failure to comply with regulatory standards can result in fines, suspension of laboratory operations, and damage to the laboratory's reputation.
The Future of Water Treatment in Laboratories
The field of water treatment in laboratories is constantly evolving, with new technologies and innovations emerging to address the growing demand for high-purity water. One of the most promising developments is the use of advanced membrane filtration technologies, such as nanofiltration and reverse osmosis, to remove a wider range of contaminants, including organic compounds and microorganisms. These technologies offer a more efficient and cost-effective way to produce high-purity water, while also reducing the environmental impact of water treatment.
Another emerging trend is the use of online water quality monitoring systems, which provide real-time data on the purity of laboratory water. These systems allow laboratories to monitor water quality continuously, ensuring that it meets the required standards and identifying potential problems early on. This can help to prevent equipment malfunctions, reduce downtime, and improve the accuracy and reliability of laboratory results. The future of water treatment in laboratories is likely to be characterized by the development of even more sophisticated and efficient technologies, further enhancing the quality and safety of laboratory water and supporting the advancement of scientific research.
Subscribe to our newsletter
Stay updated with IT-Tech Insights
Related posts
Check out other IT- Tech Scientific Resources

Troubleshooting gas leaks and pressure drops
Gas leaks and pressure drops can disrupt laboratory operations and pose safety hazards. This article outlines a systematic approach to troubleshooting these issues. It covers common causes such as faulty connections, leaks in tubing, and regulator malfunctions. The guide also emphasizes safety precautions and provides practical tips for identifying and resolving problems. By following these steps, you can ensure the integrity and reliability of your laboratory gas systems.

Backup power options for critical lab equipment
Maintaining a stable and reliable power supply is crucial for the smooth operation of critical lab equipment. Unforeseen power outages can disrupt experiments, damage sensitive instruments, and lead to costly delays. This article delves into various backup power options designed to safeguard your lab's operations and protect your research investments. We explore the advantages and limitations of UPS systems, generators, and other solutions, helping you choose the most suitable option for your specific needs and budget. By implementing a robust backup power strategy, you can minimize downtime, enhance data integrity, and ensure the continuity of your research endeavors.

Maintenance protocols for lab electrical systems
This training module provides a comprehensive overview of maintenance protocols for lab electrical systems. It covers essential topics such as routine inspections, preventative maintenance schedules, and troubleshooting procedures. The module emphasizes the importance of safety, compliance with relevant regulations, and minimizing downtime. You will learn how to identify potential hazards, perform electrical tests, and address common issues effectively. By following these protocols, you can ensure the safe and reliable operation of your lab's electrical systems, contributing to a secure and productive research environment.