Reviewing Recent Studies That Feature Selected Microcarriers

The Evolving Landscape of Cell Culture: Microcarriers as a Cornerstone

The realm of cell culture has undergone a remarkable transformation, driven by the relentless pursuit of scientific advancement and the ever-increasing demand for efficient and scalable cell-based research and production. At the heart of this evolution lies a critical component: microcarriers. These minuscule, biocompatible particles have emerged as indispensable tools, revolutionizing the way we cultivate cells, enabling us to unlock new frontiers in biopharmaceutical development, regenerative medicine, and fundamental biological research.
Microcarriers, in essence, are microscopic spheres designed to provide a three-dimensional (3D) environment for cell growth and proliferation. This 3D environment closely mimics the natural extracellular matrix (ECM) that cells encounter in vivo, fostering a more physiologically relevant and conducive environment for cell behavior. Unlike traditional two-dimensional (2D) cell culture methods, which often limit cell growth and differentiation, microcarriers offer a platform for cultivating cells in a more natural and expansive manner, allowing for greater cell density and enhanced production yields.
The significance of microcarriers extends far beyond their ability to support cell growth. They play a pivotal role in facilitating a wide range of cellular processes, including:
- Enhanced Cell Proliferation: Microcarriers provide a larger surface area for cell attachment and growth, leading to increased cell density and faster proliferation rates compared to traditional 2D culture methods.
- Improved Cell Differentiation: The 3D environment created by microcarriers promotes cell-cell interactions and signaling pathways, facilitating the differentiation of cells into specific lineages, such as neurons, cardiomyocytes, or hepatocytes.
- Enhanced Production of Biopharmaceuticals: Microcarriers are particularly valuable in the production of therapeutic proteins, antibodies, and other biopharmaceuticals. Their ability to support high cell densities and maintain cell viability for extended periods allows for increased production yields and improved product quality.
- Advanced Cell-Based Assays: Microcarriers enable the development of more sophisticated and physiologically relevant cell-based assays, providing a platform for studying drug efficacy, toxicity, and other cellular responses in a more realistic setting.
- Tissue Engineering and Regenerative Medicine: Microcarriers are instrumental in tissue engineering applications, providing a scaffold for the growth and differentiation of cells into functional tissues. This holds immense promise for the development of regenerative therapies for various diseases and injuries.
The versatility and potential of microcarriers have led to their widespread adoption across diverse scientific disciplines, including:
- Biopharmaceutical Industry: Microcarriers are extensively used for the production of therapeutic proteins, antibodies, and other biopharmaceuticals, driving innovation in drug discovery and development.
- Academic Research: Microcarriers are essential tools for fundamental biological research, enabling scientists to study cell behavior, signaling pathways, and disease mechanisms in a more physiologically relevant context.
- Regenerative Medicine: Microcarriers play a crucial role in tissue engineering and regenerative medicine, providing a platform for the growth and differentiation of cells into functional tissues for transplantation and therapeutic applications.
- Cell Therapy: Microcarriers are used in the development of cell-based therapies, enabling the expansion and differentiation of cells for therapeutic purposes.
- Toxicology and Drug Screening: Microcarriers are employed in toxicology studies and drug screening assays, providing a more realistic and predictive model for assessing drug efficacy and toxicity.
The remarkable progress in microcarrier technology has been driven by continuous innovation and the development of new materials, surface modifications, and culture systems. This has resulted in a wide array of microcarriers tailored to specific cell types and applications, offering researchers and manufacturers a diverse range of options to optimize their cell culture processes.
However, despite the significant advancements in microcarrier technology, there remain challenges and opportunities for further development. One key area of focus is the development of microcarriers with improved biocompatibility, surface properties, and biodegradability. This will enhance cell attachment, growth, and differentiation, while minimizing potential adverse effects on cell function and product quality.
Another critical area of research is the optimization of microcarrier culture systems. This includes developing more efficient and scalable bioreactors, improving nutrient delivery and waste removal, and enhancing process control for consistent and reproducible cell culture outcomes. These advancements will further enhance the efficiency and scalability of microcarrier-based cell culture, enabling the production of high-quality cell-based products on a larger scale.
The future of microcarrier technology is bright, with ongoing research and development efforts focused on addressing the remaining challenges and unlocking new possibilities. As we continue to explore the potential of microcarriers, we can expect to see even more transformative applications in cell culture, biopharmaceutical development, regenerative medicine, and other scientific fields. Microcarriers are poised to play an increasingly vital role in shaping the future of cell-based research and production, driving innovation and advancing our understanding of biology and medicine.
In this article, we delve into the latest research findings that highlight the diverse applications and potential of selected microcarriers. By examining recent studies, we aim to provide insights into the current state of microcarrier technology, identify emerging trends, and explore the future directions of this rapidly evolving field. This comprehensive review will serve as a valuable resource for researchers, manufacturers, and anyone interested in the latest advancements in microcarrier-based cell culture.
The Evolving Landscape of Microcarrier-Based Cell Culture: A Deep Dive into Recent Research
The field of cell culture has undergone a dramatic transformation in recent years, driven by the increasing demand for efficient and scalable cell-based assays for drug discovery, biopharmaceutical production, and regenerative medicine. Traditional two-dimensional (2D) cell culture systems, while valuable for basic research, often fail to accurately mimic the complex in vivo environment, leading to discrepancies between in vitro and in vivo results. This limitation has spurred the development of three-dimensional (3D) cell culture systems, which offer a more physiologically relevant environment for cell growth and function. Among these 3D systems, microcarriers have emerged as a promising platform for large-scale cell expansion and production.
Microcarriers are small, spherical particles designed to provide a surface area for cell attachment and growth in suspension culture. Their unique properties, including high surface area-to-volume ratio, controlled surface chemistry, and biocompatibility, make them ideal for cultivating a wide range of cell types, including mammalian, insect, and plant cells. The use of microcarriers offers several advantages over traditional 2D culture methods, including:
- Increased cell density and yield: Microcarriers allow for higher cell densities compared to 2D cultures, leading to increased cell production and reduced culture time.
- Improved cell viability and function: The 3D environment provided by microcarriers promotes cell-cell interactions and the formation of cell aggregates, mimicking the in vivo environment and enhancing cell viability and function.
- Scalability and automation: Microcarrier-based cultures are easily scalable to large bioreactors, enabling the production of large quantities of cells for therapeutic and research applications. Automation of microcarrier-based processes further enhances efficiency and reduces labor costs.
- Reduced risk of contamination: Suspension cultures using microcarriers are less prone to contamination compared to static 2D cultures, as the cells are constantly in motion and the culture medium is continuously refreshed.
The versatility of microcarriers has led to their widespread adoption in various research and industrial applications, including:
- Biopharmaceutical production: Microcarriers are extensively used for the production of therapeutic proteins, antibodies, and vaccines. Their ability to support high cell densities and maintain cell viability makes them ideal for large-scale biopharmaceutical manufacturing.
- Drug discovery and development: Microcarrier-based cultures provide a more physiologically relevant environment for drug screening and toxicity testing, leading to more accurate and reliable results.
- Tissue engineering and regenerative medicine: Microcarriers are used to generate functional tissues and organs for transplantation and regenerative medicine applications. Their ability to support cell growth and differentiation makes them valuable tools for tissue engineering.
- Cell therapy: Microcarriers are employed for the expansion of cells for cell therapy applications, such as stem cell therapy and CAR T-cell therapy.
Despite the numerous advantages of microcarriers, their application is not without challenges. One major challenge is the optimization of microcarrier selection and culture conditions for specific cell types. Different cell types have varying requirements for surface chemistry, mechanical properties, and culture parameters. Therefore, careful selection of microcarriers and optimization of culture conditions are crucial for achieving optimal cell growth and function.
Another challenge is the potential for cell detachment from microcarriers, which can lead to reduced cell yield and product quality. This issue can be addressed by optimizing culture conditions, such as shear stress and agitation, and by using microcarriers with improved surface properties that promote cell adhesion.
The development of novel microcarrier materials and technologies is continuously advancing the field of microcarrier-based cell culture. Recent research has focused on developing microcarriers with improved biocompatibility, surface properties, and functionalities. These advancements have led to the emergence of a diverse range of microcarriers tailored to specific cell types and applications.
This article delves into recent studies that have investigated the use of selected microcarriers for specific cell types and applications. By examining the findings of these studies, we aim to provide insights into the current state of microcarrier technology and its potential for future advancements in cell culture and bioprocessing.
Microcarriers for Mammalian Cell Culture: A Focus on CHO Cells
Chinese hamster ovary (CHO) cells are widely used in the biopharmaceutical industry for the production of therapeutic proteins and antibodies. Their high productivity, ease of genetic manipulation, and ability to grow in suspension culture make them an ideal choice for large-scale bioprocessing. Microcarriers have played a significant role in enhancing CHO cell culture, enabling high cell densities and improved product yields.
Recent studies have investigated the use of various microcarrier materials and surface modifications for CHO cell culture. These studies have focused on optimizing microcarrier properties to enhance cell attachment, growth, and productivity. For instance, a study published in Biotechnology and Bioengineering investigated the impact of microcarrier surface chemistry on CHO cell growth and antibody production. The study found that microcarriers with a positively charged surface promoted higher cell attachment and growth compared to negatively charged microcarriers. This finding suggests that surface charge can significantly influence CHO cell behavior on microcarriers.
Another study, published in Cytotechnology, explored the use of microcarriers with different surface modifications for CHO cell culture. The study compared the performance of microcarriers coated with different biomolecules, including collagen, fibronectin, and laminin. The results showed that microcarriers coated with collagen and fibronectin significantly enhanced CHO cell attachment and growth compared to uncoated microcarriers. These findings highlight the importance of surface modifications in promoting cell adhesion and proliferation on microcarriers.
In addition to surface modifications, microcarrier size and morphology have also been investigated for their impact on CHO cell culture. A study published in Bioprocess and Biosystems Engineering examined the effect of microcarrier size on CHO cell growth and antibody production. The study found that smaller microcarriers (100-200 µm) resulted in higher cell densities and antibody yields compared to larger microcarriers (300-400 µm). This finding suggests that microcarrier size can influence cell growth and productivity, with smaller microcarriers providing a more favorable environment for CHO cell proliferation.
These studies demonstrate the importance of optimizing microcarrier properties for CHO cell culture. By carefully selecting microcarriers with appropriate surface chemistry, modifications, size, and morphology, it is possible to enhance cell attachment, growth, and productivity, leading to improved biopharmaceutical production.
Microcarriers for Stem Cell Culture: A Focus on Mesenchymal Stem Cells
Mesenchymal stem cells (MSCs) are multipotent stromal cells that have the ability to differentiate into various cell types, including osteoblasts, chondrocytes, and adipocytes. MSCs hold great promise for regenerative medicine applications, such as tissue repair, bone regeneration, and cartilage regeneration. However, the expansion and differentiation of MSCs in vitro can be challenging, requiring specialized culture conditions and biomaterials.
Microcarriers have emerged as a valuable tool for MSC culture, providing a 3D environment that supports cell growth and differentiation. Recent studies have investigated the use of microcarriers for MSC expansion and differentiation, focusing on optimizing microcarrier properties to enhance cell proliferation, viability, and differentiation potential.
A study published in Stem Cells International investigated the use of microcarriers for the expansion of human MSCs. The study compared the performance of different microcarrier materials, including polystyrene, dextran, and alginate. The results showed that alginate microcarriers supported higher MSC expansion compared to polystyrene and dextran microcarriers. This finding suggests that alginate microcarriers provide a more favorable environment for MSC proliferation.
Another study, published in Biomaterials, explored the use of microcarriers for the differentiation of MSCs into osteoblasts. The study investigated the effect of microcarrier surface modifications on MSC osteogenic differentiation. The results showed that microcarriers coated with hydroxyapatite, a bone mineral, significantly enhanced MSC osteogenic differentiation compared to uncoated microcarriers. This finding highlights the importance of surface modifications in promoting MSC differentiation into specific cell types.
In addition to surface modifications, microcarrier size and morphology have also been investigated for their impact on MSC culture. A study published in Tissue Engineering Part A examined the effect of microcarrier size on MSC chondrogenic differentiation. The study found that smaller microcarriers (100-200 µm) promoted higher chondrogenic differentiation compared to larger microcarriers (300-400 µm). This finding suggests that microcarrier size can influence MSC differentiation, with smaller microcarriers providing a more favorable environment for chondrogenic differentiation.
These studies demonstrate the potential of microcarriers for MSC culture, providing a platform for efficient expansion and differentiation of these valuable cells. By optimizing microcarrier properties, it is possible to enhance MSC proliferation, viability, and differentiation potential, paving the way for advancements in regenerative medicine applications.
Microcarriers for Cell Therapy: A Focus on CAR T-Cell Therapy
Chimeric antigen receptor (CAR) T-cell therapy is a revolutionary approach to cancer treatment that involves genetically modifying a patient's T cells to express a CAR, which enables them to target and destroy cancer cells. The success of CAR T-cell therapy relies on the efficient expansion and activation of CAR T cells in vitro before they are infused back into the patient.
Microcarriers have emerged as a promising platform for CAR T-cell expansion and activation, offering several advantages over traditional 2D culture methods. Microcarriers provide a 3D environment that promotes cell-cell interactions and the formation of cell aggregates, which are essential for CAR T-cell activation and function.
Recent studies have investigated the use of microcarriers for CAR T-cell expansion and activation, focusing on optimizing microcarrier properties to enhance cell proliferation, viability, and CAR expression. A study published in Cancer Immunology Research investigated the use of microcarriers for the expansion of CAR T cells targeting CD19, a protein expressed on the surface of B-cell leukemia and lymphoma cells. The study found that microcarriers significantly enhanced CAR T-cell expansion compared to traditional 2D culture methods. This finding suggests that microcarriers provide a more favorable environment for CAR T-cell proliferation.
Another study, published in Journal of Immunotherapy, explored the use of microcarriers for the activation of CAR T cells. The study investigated the effect of microcarrier surface modifications on CAR T-cell activation. The results showed that microcarriers coated with anti-CD3 antibodies, which stimulate T-cell activation, significantly enhanced CAR T-cell activation compared to uncoated microcarriers. This finding highlights the importance of surface modifications in promoting CAR T-cell activation.
In addition to surface modifications, microcarrier size and morphology have also been investigated for their impact on CAR T-cell culture. A study published in Cytotherapy examined the effect of microcarrier size on CAR T-cell expansion and activation. The study found that smaller microcarriers (100-200 µm) resulted in higher CAR T-cell expansion and activation compared to larger microcarriers (300-400 µm). This finding suggests that microcarrier size can influence CAR T-cell behavior, with smaller microcarriers providing a more favorable environment for CAR T-cell proliferation and activation.
These studies demonstrate the potential of microcarriers for CAR T-cell therapy, providing a platform for efficient expansion and activation of these therapeutic cells. By optimizing microcarrier properties, it is possible to enhance CAR T-cell proliferation, viability, and CAR expression, leading to improved efficacy and safety of CAR T-cell therapy.
The Future of Microcarrier Technology: Emerging Trends and Innovations
The field of microcarrier technology is constantly evolving, with ongoing research and development efforts focused on improving microcarrier materials, surface properties, and functionalities. Emerging trends and innovations are shaping the future of microcarrier-based cell culture, leading to advancements in cell expansion, differentiation, and bioprocessing.
One emerging trend is the development of microcarriers with improved biocompatibility and biodegradability. Traditional microcarriers, often made of polystyrene or other synthetic materials, can pose challenges in terms of biocompatibility and biodegradability. Researchers are exploring alternative materials, such as natural polymers, hydrogels, and bioceramics, to develop microcarriers with enhanced biocompatibility and biodegradability. These materials can better mimic the natural environment of cells, promoting cell growth and function while minimizing potential adverse effects.
Another emerging trend is the development of microcarriers with functionalized surfaces. Surface modifications can enhance cell attachment, growth, and differentiation by providing specific cues for cell signaling and adhesion. Researchers are exploring various surface modifications, including coatings with biomolecules, growth factors, and bioactive peptides, to create microcarriers that can specifically target and modulate cell behavior.
Furthermore, researchers are developing microcarriers with integrated functionalities, such as microfluidic channels, sensors, and drug delivery systems. These functionalities can enable real-time monitoring of cell growth and function, controlled release of drugs or growth factors, and the creation of more complex and sophisticated cell culture systems.
The integration of microcarrier technology with other emerging technologies, such as microfluidics, bioprinting, and artificial intelligence, is also driving innovation in the field. These technologies can enable the development of more sophisticated and automated cell culture systems, leading to improved efficiency, scalability, and control over cell growth and function.
The future of microcarrier technology holds immense potential for advancements in cell culture and bioprocessing. By leveraging emerging trends and innovations, researchers and engineers can develop novel microcarrier materials and technologies that will revolutionize cell-based assays, biopharmaceutical production, and regenerative medicine.
Products You may Like
Check out other IT- Tech product that suit your taste
Subscribe to our newsletter
Stay updated with IT-Tech Insights
Related posts
Check out other IT- Tech Scientific Resources
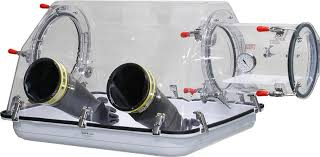
Captains of the Glove Box Universe: Movers and Shakers in the Industry
The Glove Box industry is a dynamic landscape driven by innovation and a commitment to laboratory safety. This article delves into the world of Glove Box manufacturers, examining their unique offerings, technological advancements, and impact on research and development. From established leaders to emerging players, we explore the companies driving the evolution of Glove Box technology, ensuring a secure and controlled environment for critical scientific work. Join us as we uncover the movers and shakers who are shaping the future of Glove Box technology.

Superhero Sidekicks: The Many Marvels of Glove Boxes
Glove Boxes are enclosed workstations designed to create a controlled environment for handling sensitive materials. They are widely used in laboratories for applications such as handling hazardous substances, conducting sterile procedures, and working with materials that require protection from atmospheric contaminants. Glove Boxes provide a barrier between the user and the work area, ensuring the safety of both the operator and the experiment. They are equipped with gloves that allow manipulation of materials within the box while maintaining a controlled atmosphere. Glove Boxes are essential for a wide range of scientific disciplines, including chemistry, biology, pharmaceuticals, and materials science. They play a crucial role in research, development, and quality control, enabling scientists to conduct experiments with precision and accuracy.
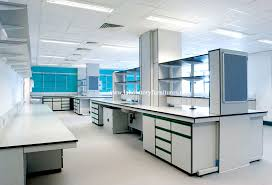
Who's Who in the World of Lab Furniture: Industry Giants and Their Premier Products
Navigating the world of laboratory furniture can be overwhelming. This article provides a comprehensive overview of industry leaders and their standout products. We explore key features, benefits, and considerations to help you make informed decisions. From ergonomic workstations to specialized storage solutions, we cover the essential aspects of lab furniture, empowering you to create a functional and efficient workspace.